NEEP533 Course Notes (Spring 1999)
Resources from Space
Lecture #27: There's Helium in Them Thar Craters!
Title: Lunar Mine Planning
The Regolith as a Mining Environment
(Please refer to Lecture 12, Stage 7: Mature Crust for other details and photographs)
Understanding the lunar regolith and obtaining quantitative data on its "geotechnical" variables will be crucial to the planning of mining operations within it and the design of hardware and software of mining and processing equipment.
Regolith: Regolith constitutes the most important material with respect to resources on the Moon. It covers most of the surfaces of the Moon and is the most logical material to process for volatiles, metals, non-metals, and aggregate. Regolith "...is a terrestrial term, also used for the Moon. It has been defined as 'a general term for the layer or mantle of fragmental and unconsolidated rock material, whether residual or transported and of highly varied character, that nearly everywhere forms the surface of the land and overlies or covers bedrock. It includes rock debris of all kinds, including volcanic ash .. '... lunar regolith consists of particles <1 cm in size although larger cobbles and boulders, some as much as several meters across, are commonly found at the surface....much of the pulverized material is melted and welded together to produce breccias (fragmental rocks) and impact melt rocks, which make up a significant portion of the regolith ..." (Heiken, et al, 1991)
Shoemaker, et al., 1968, is a particularly important reference in regard to characterization of the lunar regolith. A particularly important part of the lunar regolith consists of aggregates of rock, mineral, and glass fragments, held together by impact melt glass, called agglutinates. Further, the lunar regolith contains adsorbed solar wind gases, meteoritic material, and the products of solar and cosmic radiation. Lateral mixing of the regolith derived from various bedrock materials is a function of the age of the separating contact.
A conceptional illustration of the initial stages of regolith development is given below in cross-section. It is important to imagine the result of continuing this cratering and mixing process for nearly 4 billion years until the surface has become saturated with craters 30-50 meters in diameter. During this time, impacts of craters smaller and far more numerous than this size continually wear down excavated boulders of bedrock, impact generated regolith breccia, and the larger craters themselves. The net result is a material that is heterogeneous and incompletely mixed in detail but homogeneous over large areas and to several meters depth with respect to processing for its resources.
Conceptual Development of Lunar Regolith
in Mare Basins
This table summarizes geotechnical data necessary to plan for regolith mining operations at an optimum site on the Moon, and the source and level of our current understanding of these parameters..
This table summarizes geotechnical data necessary to design mining and processing equipment related to resources in the lunar regolith, and the source and level of our current understanding of these parameters.
Example of Mining Equipment Designed to Extract Lunar Volatiles-Example
He-3
![]() |
From lecture #19 it was shown that over 85% of the helium-3 could be removed from ilmenite by heating it to 700°C |
![]() |
The type of equipment that can accomplish the heating of large amounts of fine grain regolith is shown in the next picture. See Sviatoslavsky (1988) for more details. |
![]() |
In the process of extracting He-3, many other elements and compounds are produced. |
![]() |
The "coldness" of outer space can be used to separate the lunar volatiles. |
![]() |
The characteristics of the Mark-II miner are listed here. |
![]() |
There are at least 3 major applications for the volatile by-products from Lunar He-3 mining. |
The Life support applications were discussed in lecture 19 and a future lecture on fusion propulsion will discuss their applications in transportation. Here, we will discuss only the possibility to use the by-products for making electricity from H2-O2 fuel cells.
MORE GENERAL MINING CONSTRAINTS
Principal Mining and Beneficiation Constraints
- natural (geological) controls of resource distribution
- predictability of significant variations in host material
- location and influence of blocky-rim craters
- energy requirements to mine and process
- energy source(s) and schedule of availability
- degree of reliable automation possible
- dust control
- working fluids availability if required
- waste heat recovery technology
- applications of "shaped" explosive excavation
- equipment reliability and durability
- number and skill of human support
- waste "rock" disposal
- location of refining facility
- location of permanent support base
- transportation requirements
Principal Resource Refining Constraints
- predictability of significant variations in "concentrate"
- energy and schedule (lunar night?) requirements
- energy source(s) and schedule of availability
- degree of reliable automation possible
- equipment reliability and durability
- amount and skill of human support
- recoverable grade in fines assumed: 45 ppb (assuming that initial mine site selection has maximized recoverable grade relative to age and ilmenite abundance)
- regolith density assumed: 1.5 g/cm3
- usable regolith assumed: 50%
- Surface Bulk Tonnage Continuous Mining (similar to terrestrial bucket wheels, dredges, and draglines)
- Robotic with human intervention and maintenance
- In situ beneficiation and thermal extraction of volatiles
- Waste heat recovery
- Regolith waste disposal in mined track
- Blocky rim craters avoided to average of one crater diameter
- Craters less than 10 meters in diameter destroyed
- No major change in surface albedo expected
Regolith and Pyroclastic Volatiles Mining Approach
- Permanent Base
- Periodically Mobile Base
REGOLITH MINERALS (extraction of metals, oxygen, aggregate,
and sintering material might be combined with regolith volatiles mining)
Pre-heating accomplished
Sorting by size, shape, paramagnetism, electrostatic properties (Agosto, 1985), density, grain roughness, etc. might be inserted into processing
PRIMARY MINERALS (as layers in basalt cooling units)
Underground High Tonnage Mining (similar to terrestrial mining of underground layered deposits, e.g., coal, salt, copper, chromite, platinum, etc.)
Lava tube (NASA art S88-33546)
Mining and Sealing Concept (after Chamberlain, et al., 1993)
- Ore layer location
- impact crater mapping
- seismic and radar tomography
- drilling
- Robotic "long wall" continuous mining with close human intervention
- Existing impact breeciation may both help (pre-crushing) and hinder (caving) mining
- Continuous ore transport to surface with close human intervention
- Long term, continous movement of abrasive material on belts, in buckets, etc. will always be a challenge
- Ore preparation may make beneficiation step, below, sufficiently more efficient to be cost effective
- final crushing
- H2O, H2 and or He washing?
- surface preparation
- Surface beneficiation and physical-property-based separation
- size fraction (fine from course)
- magnetic susceptibility (ilmenite rich fines from other fines)
- density (ilmente-rich fines from other fines)
- optical properties (pyroclastic glasses and devitrified glasses)
- Waste rock disposal at surface (in craters or as mounds if not useful as aggregate for roads, etc.)
- Waste disposal will change topography
- Major changes in albedo where waste rock disposed due to grain size differences with normal regolith
POSSIBLE NEW INFORMATION REQUIREMENTS FOR OPTIMUM PLANNING AND TO MEET INVESTOR REQUIREMENTS
- Information Constraints Prior to Mining Equipment Design and Mine Planning (see tables at beginning of this lecture)
- Initial selection of most promising mine sites
- Access cost analysis for various promising areas of the Moon
- Orbital remote sensing [polar orbit satellite]
- Surface distribution to within a few meters resolution of the following:
- 3He concentration (gamma ray emission of neutron absorption)
- Blocky crater rims (high resolution photograpy)
- Ti concentration (spectural imaging)
- Three dimensional distribution (radar) to within 1/2 meter depth of the following:
- Depth of regolith
- Block concentrations
- Density
- QUESTION: WOULD FOCUSED ANALYSIS AND APPLICATION OF EXISTING LUNAR DATA BASE PROVIDE SUFFICIENT PLANNING AND DESIGN INFORMATION TO BE SUCCESSFUL AS WELL AS CONVINCE INVESTORS THAT YOU WOULD BE SUCCESSFUL?
- Surface remote sensing from automated roving platform(s)
- Instrumented to provide three dimensional in situ analysis at one or more potential mine sites as selected from analysis of remotely sensed data
- Physical and geotechnical properties
- seismic surface (R) wave tomography
- surface radar
- Chemical properties
- mass spectrometry of laser sputtered ions
- 3He in situ analysis
- mass spectrometry
- gamma ray emission of neutron absorption
- Regolith maturity
- backscatter Mossbauer Spectrometry
- Natural levels of dust and micrometeroid activity
- General
- geological analysis/mapping of walls and ejecta of craters that penetrate to various levels of the regolith
- high resolution sterro photography and optical spectrometry
- Calibration of remotely sensed data for the selected sites
- Coverage sufficient to confirm proved reserves sufficient to justify initial financial investments
Selection of first mine site if precursors are required
Mine Planning and Mining Concepts for Lunar Resources
RECTILINEAR MINING(Cameron, 1992)
General Concept of Rectilinear Mining- Mining progresses linearly, mining rectilinear blocks
- mining block size will be related to:
- detailed three dimensional character of the regolith to about 3m depth
- logistics of recovery of volatiles
- maintenance plan and schedule for miner
- All mining, beneficiation, and volatile extraction and waste heat recovery contained in miner
- Interim storage of extracted volatiles in pressurized tanks
- Tanks transported to central refining location
- Refining plant and storage and shipment facilities are part of a permanent lunar base
- Lunar base includes long duration, full service, support facilities
- New base required when mining operations reach the practical and/or economic limits of support
Cameron (1992) analyzed the resource base and the minability of Mare Tranquillitatis
Useful illustration of an early stage of mine planningLocation of Mare Tranquillitatis
- The regolith covering the 300,000 km2 of Mare Tranquillitatis is a major resource for 3He
- 28% has 20-30 wppm He
- 65% has 30-45 wppm He
Mare Tranquillitatis
Distribution of High-Ti regolith:Inferred Titanium Content of Regolith of Mare Tranquillitatis
- The most favorable area for initial mine operations is 85,000 km2 in the northeast
Rectilinear mining plan
|
Most favorable mine area |
![]() |
Amount of Minable Regolith |
![]() |
Percentage of the Total Area of Mare Tranquillitatis Occupied by Major Features |
![]() |
Minable Regolith and Helium Content of Mare Tranquillitatis |
|
400m mining blocks |
|
300m mining blocks |
![]() |
Minable percentage in relation to size of mining block |
Spiral Mining (Schmitt, et al., 1992)
Another approach to mining in cratered terrain is that suggested by circular irrigation systems

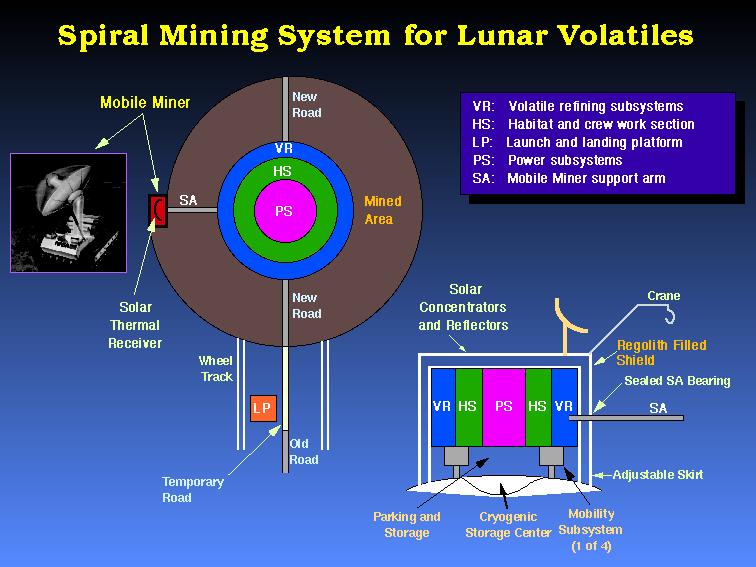
General Concept of Spiral Mining
- Mining progresses in a spiral, radially outward from a central, periodically mobile station.
- Regolith is beneficiated and volatiles are extracted and waste heat recovered in the miner
- Volatiles piped to central station for refining
Differences from Rectilinear mining- Electrical power and thermal power received from central station
- Tele-robotic operation possible along optical fiber
- Extracted volatiles pumped continuously to central station
- Refined volatiles can be shipped directly to users from central station
- Unsold volatiles can be stored under central station
- Routine maintenance and repair handled at central station
- Less demand on lunar base support means fewer or smaller lunar bases required.
Mobile Miner Characteristics:Mobile Miner Characteristics:
Central Station Characteristics:Central Station Characteristics:
Standard Duty Cycle (solar thermal only)
- daytime: mining, beneficiation, and volatile extraction
- nighttime: volatile refining (use thermal radiation to deep space)
- with sufficient storage of solar energy (H2 + O2 = H 2O cycle) or with nuclear energy production rates could be doubled)
Permanent Support Base (Logistics base)
- location should maximize access to the highest grade, lowest cost reserves and to the regional resource base
- permanent science base
- permanent settlement (?)
Requirements for planning initial mining operations Requirements for selecting initial support base site
Questions:
1. Outline the types of geotechnical (geological engineering) data that
would be required to design a Mark II-type miner.
2. Define the basic characteristics required by a cryogenic storage system
placed within the lunar regolith and how could an impact crater be adapted
to contain such a system.
3. Outline the trade-off considerations necessary to be able to choose
between rectilinear and spiral mining concepts for mining 3He
from the lunar regolith.
Text:
Cameron, 1993, pages 3 (Objectives of an Exploration Program) through end
Schmitt, et al., 1992, pages 1163-1170
Neal, et al., 1988, pages 16-18, Table 2-3
References:
Agosto, W.N., 1985, Electrostatic concentration of lunar soil minerals,in W.W. Mendel, editor, Lunar Bases and Space Activities of the 21st Century, 453-464.
Cameron, E.N., 1993, Evaluation of the Regolith of Mare Tranquillitatis
as a Source of Volatile Elements, WCSAR-TR-AR3-9301-1, 15p.
Cameron, E.N., 1992, Helium Resources of Mare Tranquillitatis, Technical
Report, WCSAR-TR-AR3-9207-1, 67p.
Chamberlain, P.G., et al., 1993, A review of possible mining applications
in space, in Resources of Near-Earth Space, edited by J. Lewis, et
al., University of Arizona Press, 51-68.
Ehricke, K. A., 1985, Lunar Industrialization and Settlement, in
W.W. Mendel, editor, Lunar Bases and Space Activities of the 21st Century,
p. 845, figure 3.
Kulcinski, G. L., Mogahed, E. A., Santarius, J. F., Sviatoslavsky, I. N., and Wittenberg, L. J., 1996, Impact of Lunar Volatiles Produced During He-3 Mining Activities, Paper AIAA-96-0490 in 34th Aerospace Sciences Meeting, january 15-18, 1996, Reno, NV.
Neal, V., et al., 1988, Extravehicular Activity at a Lunar Base, Report
on Advanced Extravehicular Activity Systems Requirements Definition Study,
NASA-17779.
Schmitt, H.H., et al., 1992, Spiral Mining for Lunar Volatiles, in
Engineering, Construction, and Operations in Space III (SPACE 92), edited
by W.Z. Sadeh, et al., v 1, 1162-1170.
Sviatoslavsky, I. N. and M. Jacobs, 1988, Mobile Helium-3 Mining System and its Benefits Toward Lunar Base Self-Sufficiency, in Engineering, Construction, and Operations in Space (SPACE 88), edited by S. W. Johnson and J. P. Wetzel, p. 310.
NEEP533 Syllabus
![]() |
University of Wisconsin Fusion Technology Institute · 439 Engineering Research Building · 1500 Engineering Drive · Madison WI 53706-1609 · Telephone: (608) 263-2352 · Fax: (608) 263-4499 · Email: fti@engr.wisc.edu |
Copyright © 2003 The Board of
Regents of the University of Wisconsin System.
For feedback or accessibility issues, contact
web@fti.neep.wisc.edu.
|