NEEP602 Course Notes (Spring 1996)
Resources from Space
Lecture #31: Opening the Solar-System Frontier
Title: Fusion Propulsion
April 10, 1996

Next:
Lecture 32: Orbiting solar satellites
Previous:
Lecture 30: Plasma and electric propulsion
Up:
Resources from Space syllabus
Developing a frontier
|
![]() From the Rheilffordd Ffestiniog Railway Web pages |
Selected events in the history of fusion for space propulsion
- 1950's to 1970's: Conceptual designs were formulated for both D-3He and D-T fusion reactors for space propulsion. These included
- Simple mirror reactors and
- Toroidal reactors with magnetic divertors
- Both type-II superconductivity and fusion power were new concepts in the early 1960's when the first D-3He design was performed.
- Three recent advances enhance the feasibility of D-3He reactors for space applications.
- Lunar 3He resources
- More credible physics concepts
- Advances in technology
Later papers, such as Roth, et al. (1972), examined the idea of adding `bucking' coils to extract a magnetic flux tube from a toroidal magnetic fusion reactor and exhaust the thrust. Although this geometry may work in relatively low magnetic field toroidal reactors, it would require massive coils and be extremely difficult for the present mainline concept, the tokamak (see lecture 26), where the magnetic fields in conceptual designs approach practical limits of about 20 T.
Arthur C. Clarke's perspective
The recognition that fusion might prove attractive for space applications was widespread. Fusion's advocates included Arthur C. Clarke, who wrote in 1961 that
Magnetic fusion fuels for space applications
Advantages of D-3He magnetic fusion for space applications
- No radioactive materials are present at launch, and only low-level radioactivity remains after operation.
- Conceptual designs project higher specific power values (1--10 kW-thrust per kg) for fusion than for nuclear-electric or solar-electric propulsion.
- Fusion gives high, flexible specific impulses (exhaust velocities), enabling efficient long-range transportation.
- D-3He produces net energy and is available throughout the Solar System.
- D-3He fuel provides an extremely high energy density.
D-3He fuel is more attractive for space applications than D-T fuel.
- High charged-particle fraction allows efficient direct conversion of fusion power to thrust or electricity.
- Increases useful power.
- Reduces heat rejection (radiator) mass.
- Allows flexible thrust and exhaust velocity tailoring.
- Low neutron fraction reduces radiation shielding.
- D-3He eliminates the need for a complicated tritium-breeding blanket and tritium-processing system.
Fusion Power Density | Neutron Power Fraction |
---|---|
![]() | ![]() |
The high fusion power density in the plasma favors D-T fuel, but the reduced neutron power fraction favors D-3He fuel. This trade-off exemplifies the competition between physics and engineering in fusion energy development. In reality, a balance among these and other considerations must be found. For space applications, D-3He fuel has usually been projected to be most attractive. The key reason for this is that the most important factor is not the fusion power density in the plasma (kW-fusion/plasma volume) but is the engineering power density (kW-thrust/mass of reactor and radiators). Several factors contribute to the dominance of D-3He fuel:
- Reduced neutron flux helps greatly
- Reduced shield thickness and mass
- Reduced magnet size and mass
- Increased magnetic field in the plasma
- Direct conversion can be used to increase the net electric power if plasma thrusters are needed.
- Many configurations can increase magnetic fields (B fields) in the fusion core, gaining power density from a B4 scaling.
B field at limit | B field near limit | Relatively low B field |
---|---|---|
Superconducting tokamak | Copper tokamak | Field-reversed configuration |
Stellarator | Heliotron | Spheromak |
Torsatron | Tandem mirror | |
Bumpy torus | ||
Reversed-field pinch |
Energy density of space-propulsion fuels
A fundamental limit on the specific power available from a fuel is the energy density of that fuel. A realistic assessment, of course, requires the detailed design of fuel storage and a means of converting fuel energy to thrust. Nevertheless, a high fuel energy density is desirable, because it facilitates carrying excess fuel, which contributes to mission flexibility, and indicates the potential for a high specific power.
The argument is often forwarded that antimatter-matter annihilation is the best space-propulsion fuel. A key difficulty exists, however: antimatter takes much more energy to acquire than it produces when annihilated with matter. Presently the ratio is about 104, and there appears little likelihood that ratios below about 102 are accessible. Antimatter, therefore, will probably be of limited use for routine access to the Solar System, although it will be the fuel of choice for specialized applications, such as interstellar missions. The energy needed to acquire various fuels is compared with the energy released in burning them in the figure at right.
High efficiency is critical in space
Fusion Reactor Designs for Space Applications
Conceptual designs of magnetic fusion reactors for space propulsion during the past decade have generally calculated specific powers of1--10 kWthrust/kgreactor. An argument supporting the general performance level, based on a `generic' analysis, appears in Santarius and Logan, 1993. The projected specific powers for selected designs appear in the table below.
NB: Widely varying assumptions and levels of optimism have gone into these conceptual designs and the resulting specific powers. For perspective, perhaps, note that my main involvement with these studies was with those that had two of the lowest specific powers listed, at about 1 kW/kg.
First Author | Year | Configuration | |
---|---|---|---|
Borowski | 1987 | Spheromak | 10.5 |
Santarius | 1988 | Tandem Mirror | 1.2 |
Chapman | 1989 | FRC | -- |
Haloulakis | 1989 | Colliding Spheromaks | -- |
Bussard | 1990 | Riggatron Tokamak | 3.9 |
Bussard | 1990 | Inertial-Electrostatic | >10 |
Teller | 1991 | Dipole | 1.0 |
Carpenter | 1992 | Tandem Mirror | 4.3 |
Nakashima | 1994 | FRC | 1.0 |
Kammash | 1995 | Gas Dynamic Trap | 21(D-T) |
Kammash | 1995 | Gas Dynamic Trap | 6.4(D-3He) |
Various fusion-reactor configurations have been considered for space applications. Generally, the key features contributing to an attractive design are
- D-3He fuel
- Solenoidal magnet geometry (linear reactor geometry) for the coils producing the vacuum (without plasma) magnetic field.
- Advanced fusion concepts that achieve high values of the parameter beta (ratio of plasma pressure to magnetic-field pressure).
Field-reversed configuration (FRC) | Tandem mirror |
---|---|
Figure to be scanned | Figure to be scanned |
Spheromak | Inertial-electrostatic Confinement |
Figure to be scanned | Figure to be scanned |
Some Inertial-confinement fusion (ICF) reactors for space propulsion have also been designed. One example is VISTA, shown at right. Because of fusion burn dynamics, D-3He fuel is much harder to use in ICF reactors, and VISTA used the D-T fuel cycle. The British Interplanetary Society's earlier Daedalus study used D-3He fuel, but had to simply assume that the physics would work. | VISTA ICF space-propulsion design |
Figure to be scanned |
D-3He Fusion Capabilities
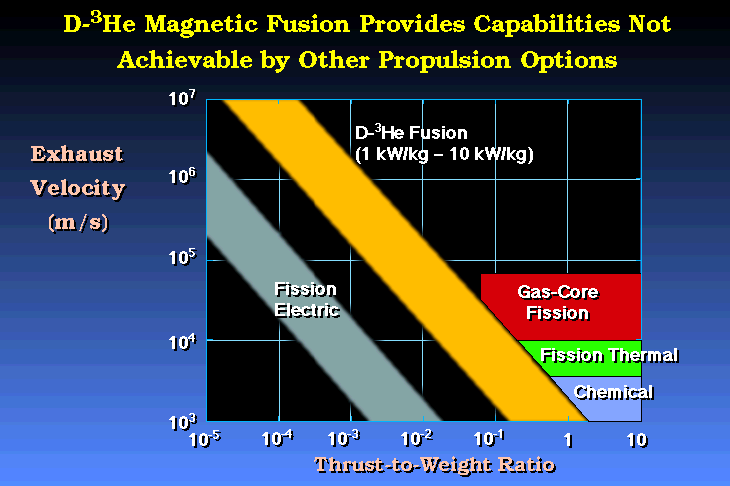
Also recall from lecture 28 that such levels of performance allow both fast human transport and efficient cargo transport, as shown in the figure at right.
Implications for space development
Although the emphasis of this document has been space propulsion, relatively small mass penalties would allow the magnetic fusion systems described here to produce electricity or to be used for materials processing by the hot fusion plasma. These applications, along with fusion's propulsion capabilities, would enable
- Human settlements on the rocky planets or in space;
- Scientific outposts near the gas-giant planets and elsewhere; and
- Access to the vast resources of the asteroids.
Useful references
Miscellaneous
- Arthur C. Clarke, ``The Planets Are Not Enough,'' in The Challenge of the Spaceship (Ballantine, New York, 1961).
- Willy Ley, Satellites, Rockets, and Outer Space (New American Library, New York, 1958), p. 17.
- Francis F. Chen, Introduction to Plasma Physics and Controlled Fusion (Plenum, New York, 1983).
- N.A. Krall and A.W. Trivelpiece, Principles of Plasma Physics (McGraw-Hill, New York, 1973).
- T. Kammash, ed., Fusion Energy in Space Propulsion (AIAA, Washington, DC, 1995).
Selected references on fusion for space propulsion
NB: Widely varying assumptions have gone into the conceptual designs presented in these papers. Proceed with caution!- A. Bond, A.R. Martin, R.A. buckland, T.J. Grant, A.T. Lawton, et al., ``Project Daedalus,'' J. British Interplanetary Society 31, (Supplement, 1978).
- S.K. Borowski, ``A Comparison of Fusion/Antiproton Propulsion Systems for Interplanetary Travel,'' AIAA/SAE/ASME/ASEE 23rd Joint Propulsion Conference, paper AIAA-87-1814 (San Diego, California, 29 June--2 July 1987).
- S.A. Carpenter and M.E. Deveny, ``Mirror Fusion Propulsion System (MFPS): An Option for the Space Exploration Initiative (SEI),'' 43rd Congress of the Int. Astronautical Federation, paper IAF-92-0613 (Washington, DC, 28 August--5 September, 1992).
- S. Carpenter, M. Deveny, and N. Schulze, ``Applying Design Principles to Fusion Reactor Configurations for Propulsion in Space,'' 29th AIAA/SAE/ASME/ASEE Joint Propulsion Conference, paper AIAA-93-2027.
- R. Chapman, G.H. Miley, and W. Kernbichler, ``Fusion Space Propulsion with a Field Reversed Configuration,'' Fusion Technology 15, 1154 (1989).
- G.W. Englert, ``Towards Thermonuclear Rocket Propulsion,'' New Scientist 16, #307, 16 (4 Oct 1962).
- J.L. Hilton, J.S. Luce, and A.S. Thompson, ``Hypothetical Fusion Propulsion Vehicle,'' J. Spacecraft 1, 276 (1964).
- T. Kammash and M-J. Lee, ``Gasdynamic Fusion Propulsion System for Space Exploration,'' J. Propulsion and Power 11, 544 (1995).
- H. Nakashima, G.H. Miley, and Y. Nakao, ``Field Reversed Configuration (FRC) Fusion Rocket,'' Proc. 11th Symp. Space Nuclear Power and Space Propulsion Systems (Albuquerque, NM, 1994).
- C.D. Orth, et al.,``The VISTA spacecraft--Advantages of ICF for Interplanetary Fusion Propulsion Applications,'' Proc. IEEE 12th Symp. on Fusion Engineering, Vol. 2, p. 1017 (IEEE, Piscataway, NJ, 1987).
- J.R. Roth, ``Space Applications of Fusion Energy,'' Fusion Technology 15, 1375 (1989).
- J.R. Roth, W.D. Rayle, and J.J. Reinmann, ``Fusion Power for Space Propulsion,'' New Scientist 54 #792, 125 (20 Apr 1972).
- J.F. Santarius, ``Magnetic Fusion for Space Propulsion,'' Fusion Technology 21, 1794 (1992).
- J.F. Santarius, ``Magnetic Fusion for Space Propulsion: Capabilities and Issues,'' Proc. Amer. Nuc. Soc. Topical Meeting on Nuclear Technologies for Space Exploration, p. 409 (Jackson, Wyoming, August 16--19, 1992).
- J.F. Santarius, ``Magnetic Fusion Propulsion: Opening the Solar-System Frontier,'' Proc. Second Wisconsin Symposium on Helium-3 and Fusion Power, p. 137 (1993).
- J.F. Santarius and B.G. Logan, ``Generic Magnetic Fusion Rocket Model,'' 29th AIAA/SAE/ASME/ASEE Joint Propulsion Conference, paper AIAA-93-2029 (Monterey, California, 28--30 June 1993).
- N.R. Schulze, ``Figures of Merit and Attributes for Space Fusion Propulsion,'' Fusion Technology 25, 182 (1994).
- E. Teller, A.J. Glass, T.K. Fowler, A. Hasegawa, and J.F. Santarius, ``Space Propulsion by Fusion in a Magnetic Dipole,'' Fusion Technology 22, 82 (1992).
Worldwide Web
Selected fusion sites
- General Atomics fusion energy educational Web site
- Lawrence Livermore National Laboratory Inertial-Confinement Fusion Web site
- Princeton Plasma Physics Laboratory fusion energy educational Web site
- University of Wisconsin Fusion Technology Institute
Questions
- Discuss the advantages and disadvantages of the key fusion fuel cycles D-T and D-3He for space applications.
- Compare the key characteristics of D-3He fusion and fission for Mars missions.
- Compare the key characteristics of D-3He fusion and fission for missions to access asteroid resources.
- Explain the main reasons that linear geometry is often preferred over toroidal geometry for magnetic fusion space propulsion.
- Discuss ICF fusion for space propulsion.



Next: Lecture 32: Orbiting solar satellites
Previous: Lecture 30: Plasma and electric propulsion
Up: Resources from Space syllabus
Dr. John F Santarius
Fusion Technology Institute,
University of Wisconsin-Madison
1500 Engineering Dr.
Madison, WI 53706
USA
415 Engineering Research Building
e-mail: santarius@engr.wisc.edu; ph: 608/263-1694; fax: 608/263-4499
Last modified: April 12, 1996
![]() |
University of Wisconsin Fusion Technology Institute · 439 Engineering Research Building · 1500 Engineering Drive · Madison WI 53706-1609 · Telephone: (608) 263-2352 · Fax: (608) 263-4499 · Email: fti@engr.wisc.edu |
Copyright © 2003 The Board of
Regents of the University of Wisconsin System.
For feedback or accessibility issues, contact
web@fti.neep.wisc.edu.
|