NEEP602 Course Notes (Fall 1996)
Resources from Space
Nuclear Power in Space
Lecture 25
Professor G. L. Kulcinski
March 25, 1996
The need for electrical energy in Space is obvious. Currently, levels of 100-500 watts are needed for small satellites while human presence will require 10's of kWe and Lunar (or Mars) bases will require 1-2 MWe. What is not so obvious is the way that this electricity will be provided. An outline of the present requirements and proposed solutions is given.
Why use nuclear energy?
For short times and low power levels, chemical or solar energy can be used to make electricity. However, at high power levels for long periods of time nuclear energy is the only way we know how to produce the necessary electrical power.
There have been at least 6 distinct driving forces behind the development of nuclear power in Space.
There are a variety of ways in which nuclear electricity can be converted to electricity.
The United States and the former Soviet Union responded to the need to develop long lasting, safe, and economical power sources in a variety of ways. However, the Russians are now only pursuing the small reactor concepts while the U. S. continues its reliance on Radioisotope Thermoelectric Generators (RTG's).
The contrast between the former Soviet Union space program and that of the U. S. is evident in the number of nuclear sources launched into orbit. Thus far, both countries have launched 86 nuclear sources into space (Bennett, 1995).
The principles of radioactive decay are quite simple.
The power generated by a RTG at any time t depends on the number of initial radioisotopes, the decay energy, and the half-life.
The radioisotope of choice is 238Pu.
Thermoelectricity is a reliable way to convert heat energy directly into electricity.
The Galileo RTG has operated perfectly.
(courtesy of General Electric Corporation)
RTG's have had a remarkable performance record (Bennett, 1995)
Why use fission reactors in Space in place of RTG's?
The release of energy from fission relies on the same conversion of mass into energy as stated by the Einstein equation.
The SP-100 nuclear reactor, designed in the U. S., would produce 100 kWe of continuous power for at least 7 years.
(courtesy of General Electric Corporation)
(courtesy of General Electric Corporation)
The SP-100 can be used for nuclear electric propulsion (see lecture 30)
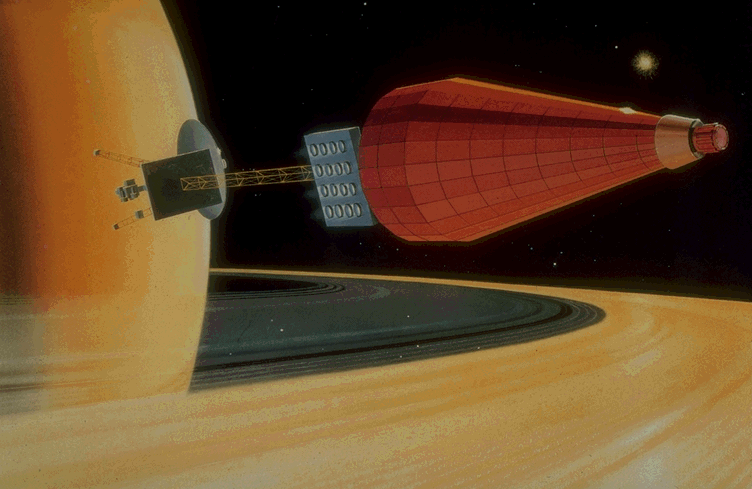
The SP-100 can be used for Base power on the Moon or Mars.
The TOPAZ-II nuclear reactor from the former Soviet Union is an improved version of the TOPAZ-I reactor which was orbited in the late 1980's.
A comparison of the different kinds of fission reactors in orbit or on the drawing board reveals their similarities and differences.
The basis for Nuclear Thermal Propulsion (NTP) can be found in the specific impulse equation.
Nuclear rockets simply provide a energy source to heat hydrogen to very high temperatures (Westinghouse, 1967).
The Nuclear Rocket Program constructed over 20 reactor cores in 16 years (Gunn, 1989).
Nuclear Rockets were developed to a high state of readiness in the 1960's (Gunn, 1989).
An artistic impression of how these NTR's might appear in Space is captured by Mr. Pat Rawlings.
(NASA artwork by Pat Rawlings/SAIC)
The achievements of the ROVER/NERVA Nuclear Rocket Program have been very impressive.
The radiation environment in Space can be very hostile.
The radiation dose to humans has been traditionally expressed in terms of Rads or Rems, by now the SI units are Grays and Sieverts.
The annual radiation dose to an average U. S. citizen is ~350 mrem.
The acute (near term) effect of radiation on humans becomes very serious as the dose exceeds several 100 Rem.
The recommended career radiation dose limits for astronauts depends on gender and age (NCRP, 1989).
Three typical NASA mission scenarios have been analyzed for radiation doses to the astronauts (NCRP, 1989).
The anticipated background radiation doses during Space missions are reasonable except for the exposure during the long trip to Mars (NCRP, 1989). Solar flares were not included and represent an additional hazard.
How much of an additional radiation dose would be experienced if nuclear power sources were used to provide the electrical energy needed on the mission or the propulsion on the trip? The answer depends on the shielding available but the additional exposure can be held to less than 10 Rem/y. In fact, if the trip time can be shortened, then the overall exposure to astronauts can be reduced by the use of nuclear power sources.
The important conclusion from this lecture is that nuclear energy will be essential to the exploration of Space by humans. The technology was developed, in some cases 20-30 years ago and will be available once we decide to go!
References
G. L. Bennett et. al., 1994, "Prelude to the Future: A Brief History of Nuclear Thermal Propulsion in the United States", p. 221 in A Critical Review of Space Nuclear Power and Propulsion, 1984-93, ed. M. El Genk, American Institute of Physics Press, New York.
Bennett, G. L. 1995, "Space Applications", CRC Handbook of Thermoelectrics, ed. D. M. Rowe, CRC Press, p. 515. See also articles by Gunn, S. V., 1989, "Development of Nuclear Rocket Technology", American Institute of Aeronautics and Astronautics Paper 89-2386
D. Buden, 1994, "Summary of Space Nuclear Reactor Power Systems (1983-1992)", p. 21 in A Critical Review of Space Nuclear Power and Propulsion, 1984-93, ed. M. El Genk, American Institute of Physics Press, New York.
National Council on Radiation Protection, 1989, "Guidance on Radiation Received in Space Activities", NCRP report #98, July 31, 1989
Westinghouse Corporation, 1967, "NRX-A6 Test Predictions", WANL-TME-1613
Representative Questions
1.) What limits the practical power level of a RTG using 238Pu?
2.) Assume you were asked to provide 500 watts of electricity and the end of life (EOL) of a 20 year mission. How much 238Pu (in kg) would you have to start with if the thermoelectrics were 4% efficient?
3.) What are the pro and con arguments for RTG's vs. small fission reactors concerning launch pad accidents?
4.) (For the Nuclear Engineers) Can you imagine why the rocket designers chose Graphite reactors over Fast Breeder reactors for a Mars mission?
![]() |
University of Wisconsin Fusion Technology Institute · 439 Engineering Research Building · 1500 Engineering Drive · Madison WI 53706-1609 · Telephone: (608) 263-2352 · Fax: (608) 263-4499 · Email: fti@engr.wisc.edu |
Copyright © 2003 The Board of
Regents of the University of Wisconsin System.
For feedback or accessibility issues, contact
web@fti.neep.wisc.edu.
|