NEEP602 Course Notes (Fall 1997)
Resources from Space
Solar Energy Resources-Lunar Power System
1) How They Work
2) Reference Design
3) Key Technology, Environmental, and Economic Issues
4) Comparisons to Other Power Sources
In contrast to the orbiting solar power satellites covered in lecture 35, the idea of placing the solar collectors on the Moon and then transmitting the microwave energy to the Earth (see Figure 1, courtesy of David Criswell) is relatively recent, i. e., since 1984.
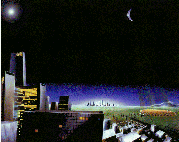
The idea was first formally proposed by Mueller (1984) and described in more detail by Waldron and Criswell (1985). However, Dr. David Criswell has been the chief architect for last decade and he has been a prolific publisher on the subject. The reader is referred to a few of his latest papers (Criswell, 1989, 1991, 1993, 1996) which contain references to over 20 of his (and co-workers) papers in the 1985-1996 period.
The reasons for pursuing the Lunar Solar Power System (LSPS) are much the same as for the Solar Power Satellites (SPS) and are listed in Figure 2. The advantages of Lunar siting, at least in the eyes of the LSPS proponents, include the stability and predictability of the Moon, abundance of all the materials needed for a LSPS, less obtrusive positioning, and better worker safety (see Figure 2).
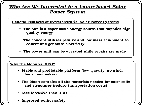
The concept proposed by Criswell has been nicely summarized by Woodcock (1989) and is described in Figure 3.
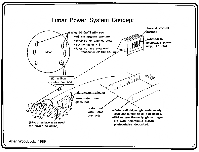
An artists conception of the Lunar base is shown in Figure 4 provided by Criswell. Note the unit in the forefront of the picture using concentrated sunlight to melt silicon for solar cells.
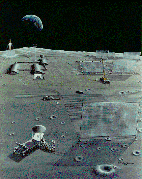
Countering the advantages of the LSPS over the SPS are the concerns listed in Figure 5.
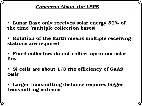
The fact that the Moon experiences a Lunar day which is approximately 14 earth days long, of course followed by a Lunar night which is also 14 earth days long, means that the solar collectors are only utilized 50% of the time. This requires that another receiving antenna be built on the opposite limb of the Moon to give constant energy to the Earth (see Figure 6).
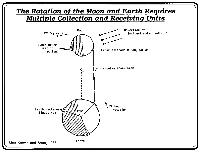
Another concern has to do with the fact that, in contrast to a GEO location where the SPS is always at a constant location to the rectenna on the Earth, there needs to be multiple receiving stations on the Earth as well. Locations at 120o apart may solve this dilemma (see Figure 6).
Other concerns have to do with the fact that fixed east-west facing solar cells on the surface of the Moon do not collect the maximum amount of energy because of shadowing during the morning or afternoon, the lower efficiency of Si cells (it is probably not cost effective to extract and make GaAs cells on the Moon), and the longer transmitting distance requires a larger transmitting antenna.
The geometric problems of a rotating Moon and Earth system can be solved by placing orbiting microwave reflectors around the Moon and around the Earth (see Figure 7).
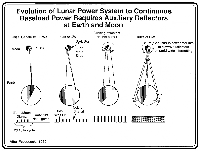
Taking all of the factors in to account it is found that a LSPS must be ~ 12 times larger than a similarly powered SPS in GEO. (see Figure 8)
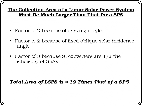
The problem of the larger transmitting antenna is illustrated in Figure 9. Assuming a 5 GWe Earth based rectenna system, the heating limits in the atmosphere dictated a 12 km diameter receiver on the Earth. A GEO-SPS system then required a 1 km diameter transmitter at 36,000 km. If the same power transmission scheme is used with same diameter rectenna receiver, then the transmitter from the Moon has to be ~ 11 km across.
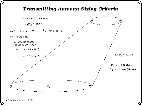
A reference design for a LSPS has been made by Criswell (1996) which is described in detail in references listed by the 1996 publication. Figure 10 is a schematic of the Lunar-Earth system which is thought to be capable of expansion to 10-20,000 GWe. The reader is strongly urged to investigate some of the previous publications to understand the level of detail with which this concept has been analyzed.
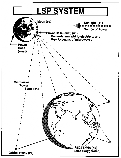
There are 3 interesting observations one can make from the LSPS reference design. First, the conversion efficiency of a lunar solar watt into electricity in the grid on the Earth is surprisingly low. Data in Figure 11, taken from Criswell (1996), shows that when the nature of the fixed E-W facing triangular Si solar cells are taken into account, only ~ 6% of the solar energy/cm2 is actually incident on the Si collectors. Since the Si converts the solar energy to electricity @ ~ 10%, less than 1% of the incident solar energy ends up as electricity on the Moon. When the conversion to microwaves, transmission to Earth based rectenna, and conversion back to electricity is accounted for, less than 0.3% of the solar energy incident on the Moon would actually end up as electricity in the Earth grid. Because of this inefficiency, it would take ~ 15% of the Moon's surface to produce 20,000 GWe on Earth, roughly all the area in the Mare of the Moon.
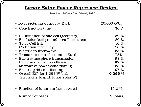
Second, in order to construct a 20,000 GWe LSPS in 30 years, ~ 2 billion tons of regolith would have to be moved on the Moon per year. (See Figure 12). The Lunar operation would require a one time mass transfer of mining equipment and support facilities to the Moon approaching 500,000 tons. This would have to be supported by a yearly transport from Earth to the Moon of ~ 250,000 tons/y. In addition, over 300,000 tons of mirrors and orbiting receivers would have to be launched from the Moon into space every year. Clearly this concept requires low cost access to space with HLV's capable of transporting 100's of tons per launch.
Third, the capital cost of the LSPS 20,000 GWe reference design approaches 22 trillion dollars (1995$) over 30 years. While such an enormous sum of money is hard to fathom, it is the order of magnitude the world will have to spend to build other electrical power producing systems (fossil, fission, fusion, etc.) if that level of power is required. It is projected that the LSPS reference concept could be built at ~1,100 $/kWe capital costs. This is roughly half the present capital cost on new electrical power systems today.
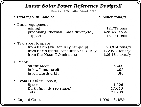
Criswell and Thompson (1996) compare the total mass/GWe for a LSPS, and terrestrial photovoltaics and terrestrial solar thermal electrical power plants. It is calculated that the LSPS design uses a factor of 6-8 less material for the same power into the Earth grid (20,000 GWe). See Figure 13.
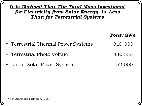
A logical question at this point is, "What if the SPS were to be made from Lunar material?" This would avoid the launch of large amounts of material from Earth to GEO and it would avoid the inefficiencies of collecting solar energy on a rotating moon. The idea was first proposed by G. O'Neill in 1974. (See Figure 14).
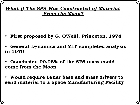
The SPS based on Lunar material was analyzed by 2 U. S. industrial firms in the mid 70's who came to the conclusion that 90-96% of the materials needed for a SPS in GEO could come from the Moon. However, this would require a substantial lunar base construction and the use of mass drivers to send the lunar derived materials to a Space Manufacturing Facility. Figure 15 contrasts the normal SPS concept with two Lunar-Derived scenarios. See Maryinak and O'Niell (1993).
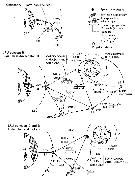
The proponents of the Lunar derived SPS claim that the launch requirements from Earth would be reduced by factors of 8-10 including the material sent to the Moon to set up the Base and the mass-launchers. This concept continues to receive attention from the Space Studies Institute in Princeton.
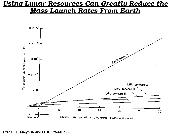
In conclusion, resources from space can be interpreted broadly to include photons or microwaves that could be converted to useful power on the Earth. There have been at least 3 major proposals to use this concept with solar energy and each has its merits and difficulties. Clearly, more detailed analysis, on a common set of assumptions (e. g., launch costs) will be required to select the most promising technology. A comparison of the optimum Solar Power Concept should then be compared to the 3He fusion concept.
References
Criswell, D. R., 1989, " Lunar Power System", p. 84 in "Report of NASA Lunar Energy Enterprise Case Study Task Force", NASA Technical Memorandum 101652.
Criswell, D. R., and Thompson, R. G., 1996, "Data Envelopment Analysis of Space and Terrestrially-Based Large Scale Commercial Power Systems for Earth: A Prototype Analysis of Their Relative Economic Advantages", Solar Energy, Vol. 56, pp. 119-131.
Criswell, D. R., 1993, "Solar Power System Based on the Moon", p. 272 in Solar Power Satellites, eds. P. E. Glaser, F. P. Davidson, and K. I. Csigi, Ellis Harwood Publ., New York,
Criswell, D. R., and Waldron, R. D., 1991, "Power Collection and Transmission System and Method", U. S. Patent 5,019,768.
Kerwin, E. M., and Arndt, G. D., 1985, "Cost Comparisons of Solar Power Satellites Versus Lunar Based Power Systems", Space Solar Power Review, Vol. 5, pp. 39-52
Maryniak, G. E., and O'Neill, G. K., 1993, "Non terrestrial Resources for Solar Power Satellite Construction", p. 253 in Solar Power Satellites, eds. P. E. Glaser, F. P. Davidson, and K. I. Csigi, Ellis Harwood Publ., New York,
Mueller, G., 1984, "The 21st Century in Space", Aerospace America, p. 84-88.
O'Neill, G. K., 1975, "Space Colonies & Energy Supply to the Earth", Science, Vol. 190, No. 4218, pp. 943-947.
Waldron, R. D., and Criswell, D. E., "Concept of the Lunar Power System", Space Solar Power Review, Vol. 5, pp. 53.
Woodcock, G. R., 1989, "Transportation and Operational Aspects of Space Energy systems", p. 97 in "Report of NASA Lunar Energy Enterprise Case Study Task Force", NASA Technical Memorandum 101652.
![]() |
University of Wisconsin Fusion Technology Institute · 439 Engineering Research Building · 1500 Engineering Drive · Madison WI 53706-1609 · Telephone: (608) 263-2352 · Fax: (608) 263-4499 · Email: fti@engr.wisc.edu |
Copyright © 2003 The Board of
Regents of the University of Wisconsin System.
For feedback or accessibility issues, contact
web@fti.neep.wisc.edu.
|